Error localization method of structural modeling based on equivalent element modal energy cha
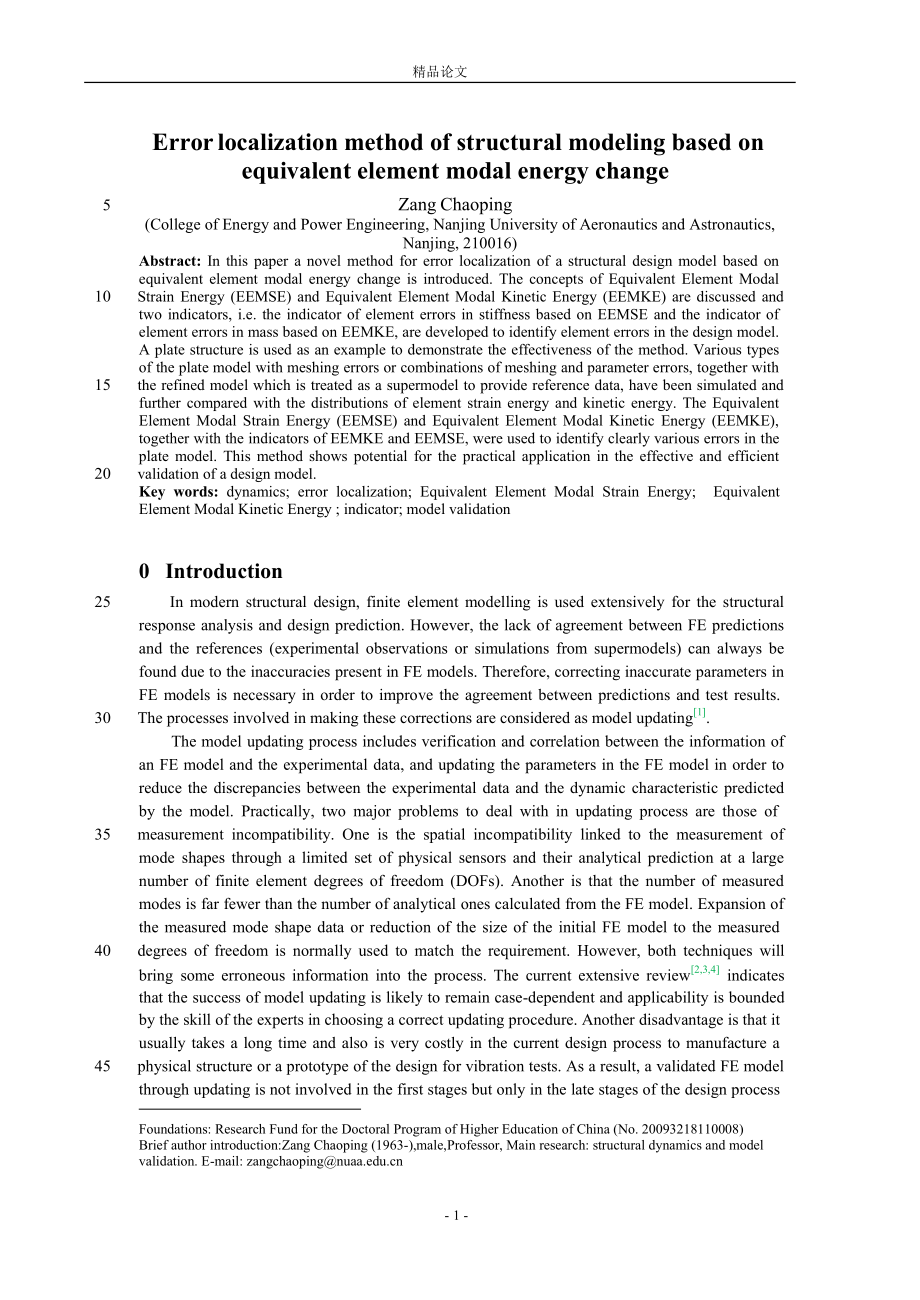


《Error localization method of structural modeling based on equivalent element modal energy cha》由会员分享,可在线阅读,更多相关《Error localization method of structural modeling based on equivalent element modal energy cha(15页珍藏版)》请在装配图网上搜索。
1、精品论文Error localization method of structural modeling based on equivalent element modal energy change5Zang Chaoping(College of Energy and Power Engineering, Nanjing University of Aeronautics and Astronautics, Nanjing, 210016)Abstract: In this paper a novel method for error localization of a structura
2、l design model based onequivalent element modal energy change is introduced. The concepts of Equivalent Element Modal10Strain Energy (EEMSE) and Equivalent Element Modal Kinetic Energy (EEMKE) are discussed and two indicators, i.e. the indicator of element errors in stiffness based on EEMSE and the
3、indicator ofelement errors in mass based on EEMKE, are developed to identify element errors in the design model.A plate structure is used as an example to demonstrate the effectiveness of the method. Various types of the plate model with meshing errors or combinations of meshing and parameter errors
4、, together with15the refined model which is treated as a supermodel to provide reference data, have been simulated and further compared with the distributions of element strain energy and kinetic energy. The EquivalentElement Modal Strain Energy (EEMSE) and Equivalent Element Modal Kinetic Energy (E
5、EMKE), together with the indicators of EEMKE and EEMSE, were used to identify clearly various errors in theplate model. This method shows potential for the practical application in the effective and efficient20validation of a design model.Key words: dynamics; error localization; Equivalent Element M
6、odal Strain Energy;EquivalentElement Modal Kinetic Energy ; indicator; model validation0Introduction25In modern structural design, finite element modelling is used extensively for the structural response analysis and design prediction. However, the lack of agreement between FE predictions and the re
7、ferences (experimental observations or simulations from supermodels) can always be found due to the inaccuracies present in FE models. Therefore, correcting inaccurate parameters in FE models is necessary in order to improve the agreement between predictions and test results.30The processes involved
8、 in making these corrections are considered as model updating1.The model updating process includes verification and correlation between the information of an FE model and the experimental data, and updating the parameters in the FE model in order to reduce the discrepancies between the experimental
9、data and the dynamic characteristic predicted by the model. Practically, two major problems to deal with in updating process are those of35measurement incompatibility. One is the spatial incompatibility linked to the measurement of mode shapes through a limited set of physical sensors and their anal
10、ytical prediction at a large number of finite element degrees of freedom (DOFs). Another is that the number of measured modes is far fewer than the number of analytical ones calculated from the FE model. Expansion of the measured mode shape data or reduction of the size of the initial FE model to th
11、e measured40degrees of freedom is normally used to match the requirement. However, both techniques will bring some erroneous information into the process. The current extensive review2,3,4 indicates that the success of model updating is likely to remain case-dependent and applicability is bounded by
12、 the skill of the experts in choosing a correct updating procedure. Another disadvantage is that itusually takes a long time and also is very costly in the current design process to manufacture a45physical structure or a prototype of the design for vibration tests. As a result, a validated FE model
13、through updating is not involved in the first stages but only in the late stages of the design processFoundations: Research Fund for the Doctoral Program of Higher Education of China (No. 20093218110008) Brief author introduction:Zang Chaoping (1963-),male,Professor, Main research: structural dynami
14、cs and model validation. E-mail: zangchaoping- 15 -and the effectiveness of its guiding of the design is extremely limited.Recently, a new method of using simulations from supermodels 5, 6 is developed to replace the experimental data as a reference for model updating. A supermodel is considered to
15、be capable50of representing all geometric features and its dynamic properties and, therefore, can be taken as representative of those of the actual structure. As a supermodel is usually created with a refined mesh and can be ideally employed in virtual experiments, it would obviously be advantageous
16、 to help to construct and update the design model. In a model updating process, based on a supermodel, more modes can be produced and more accurate mode shapes can be represented by55as many DOFs as required. Such features can overcome the drawback of measurement incompleteness and generally avoid i
17、ll-conditioning problems in updating solutions. However, the method for updating-parameter selection based on reference information from supermodels still needs to be explored. Currently, the selection of updating parameters is generally based on the engineers judgment and experience. The sensitivit
18、y matrix in iterative updating methods can help60to select the updating parameters. However, the parameters to be selected only indicate those with high sensitivity and not those with erroneous values. In this paper, a new method to localise errors in the design model for model updating via supermod
19、els will be presented and explored.1Error localization of a model based on equivalent element modal energy change651.1Overview of modeling errorsA design model, usually with a course mesh, is considered to include three kinds of error7: (1) discretisation errors, (2) configuration errors, and (3) pa
20、rameter errors. Discretisation errors are those that arise because the mass and stiffness matrices for each element of the model are formed in a way that is different from the equation of motion for a continuous system. Configuration70errors are those that arise because some physical features are om
21、itted when model simplifications are made in representing complicated parts in a structure. Correction of discretisation and configuration errors is normally involved in the scope of design updating. Parameter errors are those that result from inaccurate estimation for some parameters while construc
22、ting the model. In the updating process, such errors can be corrected in order that the coefficients in the model are75sufficiently accurate to enable the model to provide an acceptably correct description of the subject structures behaviour. As all three types of error are mixed in a design model,
23、a big challenge is to recognise them properly and find the causes of the discrepancies in the model.1.2Concepts of element strain energy and element kinetic energyFor a discrete finite element system, the dynamic equation of an element can be expressed as80me u&e + ce u& e + k e ue = fe (1)where ue
24、is the vector of generalized nodal displacements which may include translationaldisplacements, rotational displacements or other quantities.fe is the equivalent nodal forcevector of externally-applied forces. Matrices ke ,ce ,and me are defined as the elementstiffness, damping, and mass matrices res
25、pectively. If the relationship between the element nodal85displacement ue and the system (or global) nodal displacements are established throughgeometrical relations as below:where Te ue = Te is a transformation (or connectivity) matrix for element e.(2)Then, the element strain energy (ESE) can be g
26、iven byESE = 1 u T ku = 1 T T T kT e 2 e e e 2e e ee= 1 T K902(3)where K = T T kT which is the element stiffness matrix associated with the globale e e enodal displacement vector .Similarly, the element kinetic energy (EKE) can be given byEKE = 1 u&T m u& = 1 & T T T m T & e 2 e e e 2e e ee= 1 & T M
27、 & 2 (4)e e e e95where M = T T m T displacement vector .is the element mass matrix associated with the global nodal1001.3Element modal strain energy and element modal kinetic energyFor each element of the finite element model, Equations (3) and (4) show the element strain and kinetic energy under th
28、e equivalent nodal force vector of the externally applied forces. Both energies include the contribution of all vibration modes excited by the externally applied forces in dynamics.In order to investigate the elemental strain energy and kinetic energy caused by each mode, the Element Modal Strain En
29、ergy (EMSE) and the Element Modal Kinetic Energy are introduced. Considering the jth element for contribution of the ith mode, the Element Modal StrainEnergy (EMSE) and the Element Modal Kinetic Energy (EMKE) can be written asEMSE= 1 f T K f105ij 2 ie j i(5)1 & T &1 2 T MEMKEij = 2 f ie j f= wi fMi
30、2 ie j f i(6)jwhere Ke is the jth element stiffness matrix andM e is the jth element mass matrix.wiji2 is the eigenvalue of the ith mode of the structure and fis the associated global nodal110115displacement of the ith mode corresponding tothe jth element.1.4Equivalent element modal strain energy an
31、d equivalent element modal kinetic energyThe EMKE and EMSE of a mode in Equations (5), and (6) can tell the energy distribution over all the elements. As the energy distribution of a model is calculated using its own dynamic features (eigenvalues and eigenvectors), direct comparison of the EMKE and
32、EMSE distributions of two models (for example, a design (coarse) model and a supermodel (refined model) may not able to straightforward describe the source of the discrepancies between them. It is because that the EMKE and EMSE of supermodel and design model are calculated independently. To build a1
33、20relationship between two models, a new method will be developed to calculate the Equivalent Element Modal Strain Energy (EEMSE) and the Equivalent Element Modal Kinetic Energy (EEMKE) using the modal data of the eigenvalues and eigenvectors from the supermodel and the element stiffness matrices an
34、d mass matrices from the design model.The equation of calculatingthe Equivalent Element Modal Strain Energy (EEMSE) is written as1 s T d s EEMSEij = 2 fi Ke j f i(7)125And the equation of calculating the Equivalent Element Modal Kinetic Energy is expressed as1 &s T d &s 1 ( s )2 s T d s EEMKEij = 2
35、fi Me j f= wii 2f i Me j f i(8)whereK d is the jth element stiffness matrix of a design model ande jsM d is the jthejelement mass matrix of a design model. wiis the eigenvalue of the ith mode of the supermodeliand f s is the associated global nodal displacement of the ith mode of the supermodel13013
36、5corresponding to the jth element of a design model.1.5Indicators of error localizationBased on the definitions of the Equivalent Element Modal Strain Energy (EEMSE) and the Equivalent Element Modal Kinetic Energy (EEMKE), two indicators are proposed in order to localise possible discrepancies in a
37、design model.The first indicator is called the indicator of Equivalent Element Modal Strain Energy that aims to localise the possible errors in the elemental strain matrix of a design model. The second indicator is the indicator of Equivalent Element Modal Kinetic Energy that indicates possible erro
38、rs in the elemental mass matrix of a designmodel. Both indicators are written ascstiffness =EEMSE d - EMSE sij ijEMSE scmass =ijEEMKE d - EMKE sijijEMKE s(9)140ij (10)ijwhereEEMSEdis the equivalent element modal strain energy of the jth element of the designmodel on the corresponding ith mode of the
39、 supermodel.ijEEMKEdis the equivalent elementmodal kinetic energy of the jth element of the design model on the corresponding ith mode of theijsupermodel.EMSE sis the element modal strain energy of the ith mode of the corresponding145region of the supermodel matching or approximately matching the sa
40、me area of an jth individualijelement of the design model.EMKEsis the element modal kinetic energy of the ith mode of150the corresponding region of the supermodel matching or approximately matching the same area of an jth individual element of the design model. Theoretically, the indicators will equ
41、al to zero if there is no discrepancies between the design model and the supermodel. When the discrepancy errors exist in the model, the values of indicators will describe the relative differences of energy distribution between two models compared with the energy distribution of the supermodel, whic
42、hindirectly indicate the mass or stiffness difference between both models2Model validation with a plate structure1551601651701751802.1ConfigurationThree FE models of a plate structure, which are Models A, B, and C, with the same dimensions are built and illustrated in Figure 1.Model A and B are crea
43、ted with the same mesh of 24 elements and 39 nodes and Model C with refined mesh of 384 elements and 441 nodes. In addition, material and property errors in some elements were added in Model B. The value of Youngs Modules in element 2 was increased by 50% while the value in element 18 was reduced by
44、 30%. In the same time, the values of mass density in element 12 and 18 were reduced by 20%. The refined model C is considered as the supermodel and is used as a reference and the coarse model A and model B are treated as the design models. Model B also includes the mixture of discrepancy errors cau
45、sed by the mesh and parameter errors.Fig. 1 FE models of a plate structureThe correlations of Models A and B against Model C were undertaken respectively. The natural frequency differences and the MAC values are listed in Tables 1 and 2. Notes in both tables describe the actual mode shapes of the mo
46、de. For instance, ZB1 refers to the first bending mode in the z direction. ZT2 refers to the second torsion mode in the direction and YB2 refers to the second bending mode in the y direction, etc.From the comparison in Tables 1 and 2, we can see the following features:lAll the modes in Models A and
47、B are correlated with the modes in reference Model C.Modes 11 and 12 in Models A and B, compared with the modes in Model C, swapped with each other.lAll the NFD parameters between the predicted models (Model A and Model B) and the reference model (Model C) are very small excluding the CMP modes 10,
48、which are 9% between Model A and Model C and 10%, between Model B and Model C.lThe diagonal values of MAC tables are both close to unit, although the parameter errors in Model B results in a slight decrease in MAC values.Tab. 1 Correlations between Model A and Model C of the plate structureModel AMo
49、del CMODEFREQ/HzMODEFREQ /HzMACERROR/%Notes13.35613.355100.00.ZB1221.171220.989100.01.ZB2332.444332.301100.00.YB1432.862432.786100.00.ZT1560.399558.811100.03.ZB36100.6806100.180100.00.ZT27121.9307115.420100.05.ZB48175.1308172.830100.01.ZT39179.8509174.940100.03.YB210209.37010191.00099.99.ZB511257.83
50、012257.480100.00.X112261.70011253.700100.03.ZT4Tab. 2 Correlations between Model B and Model C of the plate structureModel AModel CMODEFREQ/HzMODEFREQ /HzMACERROR/%Notes13.47013.355100.03.ZB1221.435220.98999.92.ZB2333.470332.301100.03.YB1433.539432.78699.92.ZT1560.968558.81199.94.ZB36103.3406100.180
51、99.73.ZT27122.7407115.42098.86.ZB48176.5008172.83099.72.ZT39182.1909174.94099.94.YB210211.67010191.00099.610.ZB511262.47012257.48099.92.X112266.83011253.70099.75.ZT41851901952.2EMKE and EMSE of plate structuresTo localise the differences among 3 models, the EMKE and EMSE of the modes are calculated
52、and compared. The values of EMKE and EMSE of the modes 1, 3, and 12 are plotted in Figures 2, 3 and 4. Actually, Mode 3 is the first bending mode in the y direction and Mode 12 is the first mode in the x direction. Therefore, Mode 1, 3, and 12 are the first modes in the x, y, z directions. As can be
53、 seen from the plots, the values of EMKE and EMSE of the design model (the coarse model), that is, EMKE_d, and EMSE_d , are perfectly matched with those of the reference model ( the supermodel) , i.e, EMKE_s, and EMSE_s. This means that there are no parameter errors between the coarse model A and th
54、e reference Model C and the discretisation errors caused by the different meshing qualities can also be ignored for these 3 modes. Meanwhile, the difference of EMKE and EMSE of these modes between the design Model B (EMKE_er, and EMSE_er ),which includes some parameter errors,and the Models A and C
55、are clearly seen, but there are no indication that where the errors located in the Model B. It is because the discrepancies in the eigenvalues and eigenvectors of Model B resulted from the parameter errorswill be distributed among all DOFs of the model.200Fig. 2 Comparison of the EMKE and EMSE of th
56、e 1st mode among the models A, B, and C.Fig. 3 Comparison of the EMKE and EMSE of the 3rd mode among the models A, B, & C205Fig. 4 Comparison of the EMKE and EMSE of the 12th mode among the models A, B, & CThe same results can be seen in Figures 5, 6 and 7, which show the distribution of EMKE andEMS
57、E of the modes 2, 6, and 9 respectively, although there are slight disagreements in strain and kinetic energy distribution between Model A and Model C.210Fig. 5 Comparison of the EMKE and EMSE of the 2nd mode among the models A, B, & CFig. 6 Comparison of the EMKE and EMSE of the 6th mode among the
58、models A, B, & CFig. 7 Comparison of the EMKE and EMSE of the 9th mode among the models A, B, & C2152202252.3EEMKE and EEMSE of plate structuresTo localise the errors in the model, the Equivalent Element Modal Kinetic Energy (EEMKE) and the Equivalent Element Modal Strain Energy (EEMSE) are calculated using the information of eigenvalues and eigenvectors of the modes from a reference model (supermodel) and data of the element stiffness and mass matrixes of a
- 温馨提示:
1: 本站所有资源如无特殊说明,都需要本地电脑安装OFFICE2007和PDF阅读器。图纸软件为CAD,CAXA,PROE,UG,SolidWorks等.压缩文件请下载最新的WinRAR软件解压。
2: 本站的文档不包含任何第三方提供的附件图纸等,如果需要附件,请联系上传者。文件的所有权益归上传用户所有。
3.本站RAR压缩包中若带图纸,网页内容里面会有图纸预览,若没有图纸预览就没有图纸。
4. 未经权益所有人同意不得将文件中的内容挪作商业或盈利用途。
5. 装配图网仅提供信息存储空间,仅对用户上传内容的表现方式做保护处理,对用户上传分享的文档内容本身不做任何修改或编辑,并不能对任何下载内容负责。
6. 下载文件中如有侵权或不适当内容,请与我们联系,我们立即纠正。
7. 本站不保证下载资源的准确性、安全性和完整性, 同时也不承担用户因使用这些下载资源对自己和他人造成任何形式的伤害或损失。