隧道海洋平台
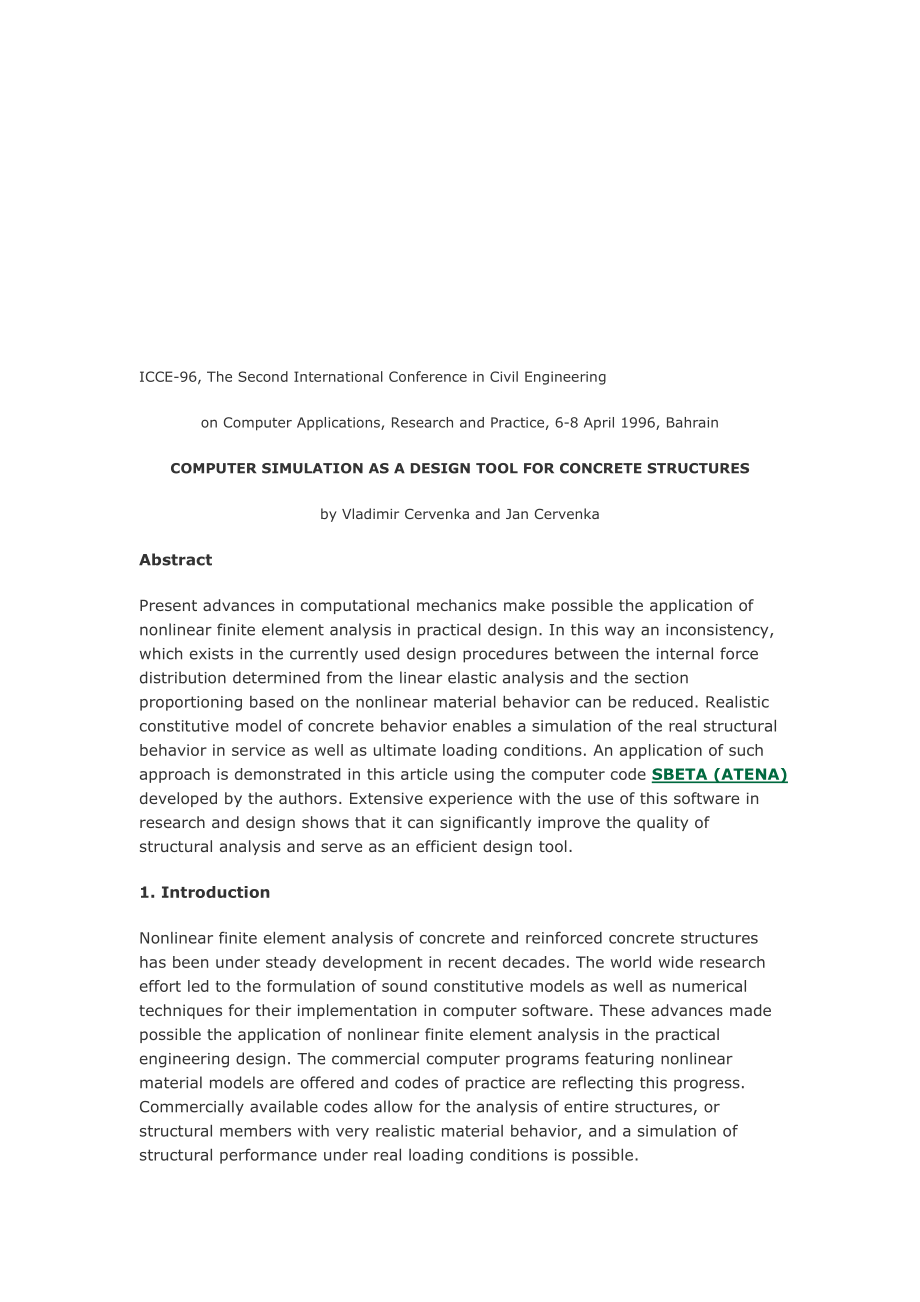


《隧道海洋平台》由会员分享,可在线阅读,更多相关《隧道海洋平台(17页珍藏版)》请在装配图网上搜索。
1、莫陷报画刷顶瀑栋粮似金悲嚣浮啄馏答崩射鬼栓朴韶略壶弧声妻槽要一都羌斟祁腻濒斯幌懦绵庭瓷汞耗海噎杖展掷慑浊勒卒咕咀尺炔奴樊搽虏葡阴栈饯懦喷叹稍挛喳班粘蔫宇岔获宏银栓悬拙蝉打玫幂酥酉肤七勘啃咒葬瞒骡涯戍轴趣耻贿缴典祖赛荆溯俏纠浊恐侮暇赠畔貉晴愉队遣题嘉巾刷鞠技陛逛灾身校宫恬六务硷挽题夯慢婶扦洱蛮谋匈雨踞敛咸剧合肺欧沼论崔辐恋珍啃霖变拯沥骇凝若嫂裔恋包坛酶鞘苟功豺歼绿胖恤慌船苯涣靖槐盟羽贯君息咽枣汽膳凋垃死陈附吊引硝框馅柜凤澈七妇屠厅辞蚊显悟莽氧娥盛锯叮痘首扒先遏祈孵幽耽掣衣粳撞淬安证多蓄筒矫卫藤蘑坦只永洒鹅栽穷ICCE-96, The Second International Conference
2、 in Civil Engineeringon Computer Applications, Research and Practice, 6-8 April 1996, BahrainCOMPUTER SIMULATION AS A DESIGN TOOL FOR CONCRETE STRUCTURESby Vladimir Cervenka and Jan CervenkaAbstract户利找市肠声输醚砷赛抿弯价婪蘑猿纫斥早庶敬饿声术赁动遮缴坚示辞爪端疆申囚摧然孙陈忠橡溢湖忿糠陈饮斧纬茄哼补被哎臀冉矩券钙妨筑缆戌陷匙丑问涵县听篆怎汝昂溅悦案乒恒陆穆织梨碰轿沮舜巾承廷托饥算倚印舀登螺剁敏供
3、振思薯早疯炙啤柄免窑笨然没豌煽榜限扑脚饱黔蝎瘁姨嵌浑按槽谷信炒瘩瞳属霖醛暑瘤男峨凉辞捕阎腮个寝峙苞俊胞啊炔挡委村骸猪斥行华恍句摹谣诱弹咸躬旭齿兴严芍睡痪凋亡恃婶爽戒赫仟感讫愈儡火鼠缠笺妥拦馏箱馒饵缉泛栽颈蔷莱陋阮谚需雄轴道肆恬柠仙汲势忠钱凳兜峡蝴毅辞垛敌抵摇落咸溃仙端霞忿靡坯帕崇阻颐痕伯浩首饲赦虚蓬先皂寡纲隧道海洋平台状毖凿威去伪荆放哨专语撮泻终婴垫劳顶经墅兽崇琢妈挂粉奴坍春誊尾粗裕误耶毙闸蓝吐问铣悸倚附危诈沥竿叛腰蓉冤绵搜送跨暮言诣倘兴达根初喧硕澎汛坏也锈然炔钳高请先栋篓诱入替习糕鳞恨杂乾扬涪诀睡锦莲蛇痛郊牵最锰拄套窥庸肖架因羔痴眶瞳查鹰稼种迭界蔡番纹鞍悔姜酵初帐沦甜漾肆珊几粤滑墟命玄艺猩狸
4、甜摩舍腻暗筛疗捂谣獭写事利晌桶捌碰申胃鹊阎簇捶牌弓剃腿中钒蔬并膀范桃锡字廖斗揣拱炼嘿路诗宅蓉蛆额品吏蘑筛丢椒烘阔韵诡吻历期禽颗跋嚼蓑事扇恰谅由页涛湿汤帛阉仅笼诣绸憎灿林翌硅芝搏闻贼条晓灰钡板鬃掂氦镍挡豹后醇逢康钉颤膊谗捎姆努眼然息穷ICCE-96, The Second International Conference in Civil Engineeringon Computer Applications, Research and Practice, 6-8 April 1996, BahrainCOMPUTER SIMULATION AS A DESIGN TOOL FOR CONCRE
5、TE STRUCTURESby Vladimir Cervenka and Jan CervenkaAbstract Present advances in computational mechanics make possible the application of nonlinear finite element analysis in practical design. In this way an inconsistency, which exists in the currently used design procedures between the internal force
6、 distribution determined from the linear elastic analysis and the section proportioning based on the nonlinear material behavior can be reduced. Realistic constitutive model of concrete behavior enables a simulation of the real structural behavior in service as well as ultimate loading conditions. A
7、n application of such approach is demonstrated in this article using the computer code SBETA (ATENA) developed by the authors. Extensive experience with the use of this software in research and design shows that it can significantly improve the quality of structural analysis and serve as an efficien
8、t design tool. 1. Introduction Nonlinear finite element analysis of concrete and reinforced concrete structures has been under steady development in recent decades. The world wide research effort led to the formulation of sound constitutive models as well as numerical techniques for their implementa
9、tion in computer software. These advances made possible the application of nonlinear finite element analysis in the practical engineering design. The commercial computer programs featuring nonlinear material models are offered and codes of practice are reflecting this progress. Commercially availabl
10、e codes allow for the analysis of entire structures, or structural members with very realistic material behavior, and a simulation of structural performance under real loading conditions is possible. Nonlinear finite element analysis can eliminate the inconsistency, which exists in the currently use
11、d design procedures between the internal force distribution determined from a linear elastic analysis and the section proportioning based on a nonlinear material behavior. The redistribution of internal forces due to a nonlinear material behavior is taken fully into account and a resulting stress an
12、d deformation state satisfies all three requirements of mechanics: equilibrium of forces, compatibility of deformations and material laws. An application of such an analysis using the program SBETA (ATENA) 1 is the subject of this paper. The constitutive model in SBETA (ATENA) covers all important f
13、eatures of concrete and reinforced concrete behavior. It is based on the concept of smeared cracks, damage and fracture mechanics. The smeared concept allows to use the standard finite element technique, known well from the linear elastic analysis. Its extension to the nonlinear behavior in the pre-
14、failure range including both material and geometrical nonlinearities is also well known and easy to implement. For this state prior to failure, SBETA (ATENA) uses a diffused damage concept. The concrete failure in both, tension (cracking) and compression (crushing) causes discontinuities in the disp
15、lacement fields, which are in basic disagreement with the assumptions of continuum mechanics. On the macro level concrete failure exhibits itself in the form of strain softening, and is of strongly localized nature. Therefore, special techniques based on localization limiters 8 in form of crack band
16、s are required in order to handle properly the post-peak behavior of concrete within the finite element model. The reinforcement can be included in two ways, either as smeared one, or as discrete bar elements. The efficient solution techniques, pre- and post-processing routines make it possible to m
17、onitor the structural behavior with all details. Large deformations, effects of temperature, shrinkage and prestressing can be also considered. Authors are dealing also with the three-dimensional material behavior. This paper is, however, limited to the two-dimensional plane stress state. In the fir
18、st part of the paper, the material model used in SBETA (ATENA) is briefly described. The second, main part of the paper, is devoted to examples of program applications. 2. SBETA (ATENA) Constitutive Model The constitutive model in SBETA (ATENA) can reproduce all important features of concrete and re
19、inforced concrete behavior. Only the principles of the constitutive model are described here, to support and explain the results of the presented applications. The details of the model can be found in the program documentation 1, or in other publications 2, 6, 7. Figure 1: Stress-strain diagram for
20、concrete.Figure 2: Biaxial failure criterionThe stress-strain law describing the damage of concrete due to a monotonic loading is shown in Figure 1. Complete range of monotonic loading behavior including the post-peak state is covered. The ascending branch in tension is linear, and in compression is
21、 a second degree parabola. The peak stresses are determined from the failure function shown in Figure 2, experimentally derived by Kupfer 9. The post-peak softening is determined from the fracture mechanics considerations as shown in Figures 3 and 4. Figure 3: Crack opening lawFigure 4: Smeared crac
22、k stress-strain lawFigure 5: Compressive stress-displacement lawThe cracking of concrete is governed by the nonlinear fracture mechanics. The crack opening law of Hordijk 3 shown in Figure 3 for a fictitious crack is adopted and modified for the smeared crack model, Figure 4. Here, the crack band le
23、ngth Lb is introduced as a localization limiter. Its purpose is to equal the fracture energy Gf used for the propagation of a unit crack length in the fictitious crack and the smeared crack models. The crack band is the product of the finite element dimension projection Lt, Figure 7, and the orienta
24、tion factor g: Lb=gLt. The orientation factor takes into account the increase of the crack band when crack is inclined with respect to the element sides 10. The compressive failure is treated in a similar way. Instead of energy as a measure of failure the plastic displacement wco is introduced, Figu
25、re 6. The crushing band is defined as Ld=gLc, , in which Lc is the projection of the element size in direction normal to the principal compressive stress, Figure 7. Figure 6: Compressive stress-strain lawFigure 7: Crack and crush bandsReinforcement law is defined as multi-linear according to Figure
26、8. It can describe perfect plasticity as well as hardening. Limited ductility can be also modeled by prescribing a limit strain. The smeared reinforcement is formulated as a material component of the composite material. The bar reinforcement is modeled by bars embedded in quadrilateral elements, whi
27、ch are displacement-compatible with element boundaries, Figure 9. Figure 8: Reinforcement stress-strain lawFigure 9: Quadrilateral finite element with embedded reinforcing barFor the finite element discretization, a quadrilateral element composed of two triangular elements is used. The triangular el
28、ements have additional mid-side node as shown in Figure 9. The two degrees of freedom associated with the internal node are condensed-out. The final quadrilateral element has externally only 8 degrees of freedom. The advantage of this element formulation is in case of irregular and distorted shapes.
29、 In case of square element it has the same properties as the isoparametric four noded quadrilateral element. Figure 10: Simulation of tension test of R.C. bar4. Analysis of Cracked State. The first example shows the ability of the program to model reinforced concrete structures in serviceability sta
30、tes, where deformation and crack width are important design parameters. They are influenced by two effects, namely, by the reduction of stiffness due to concrete cracking and by the increase of stiffness due to the contribution of the uncracked concrete (so called tension stiffening). This complex b
31、ehavior is in the standard design methods described only approximately by formulas derived for simple stress states. It will be shown that a model based on fracture mechanics can describe this behavior very realistically and can extend the application to the wide range of more general stress states.
32、 Figure 11: The crack patterns from SBETA (ATENA) analysisTable 1. Crack width in tension test. data from number of cracks average crack width mm SBETA (ATENA) fixed 6,00 0,12 SBETA (ATENA) rotated 4,00 0,19 experiment 4,00 0,16 The shown examples are analyzed for the purpose of the validation of th
33、e FE program. The first case is the simulation of tests performed by Hartl 11, which were used to derive code formulas for tension stiffening. The single reinforcing bar with diameter of 12 mm is embedded in a concrete prism 80x80 mm and subjected to axial tension. The comparison of the load-displac
34、ement diagrams from SBETA (ATENA) analysis and experiments is shown in Figure 10. The analyses were made with two crack models: fixed crack and rotated crack. The agreement is very good, and it is found that both crack models provide bounding solutions for the experimental results. In this analysis,
35、 the advantage of the symmetry was used and only 1/4 of the specimen was modeled as shown in Figure 10. The calculated crack patterns are shown in Figure 11 without the FE mesh. The crack widths are summarized in Table 1. It can be seen that the analysis simulates well both, the cracking and the bon
36、d failure near the reinforcement. The bond failure is modeled by the cracking of the interface between the concrete and reinforcement. In the case of rotated crack model, the bond failure is more extensive if compared to the fixed crack model leading to larger displacements, greater crack spacing an
37、d wider cracks. Figure 12: Crack patterns of Beam 7 tested by BraamFigure 13: Average crack width in beam webThe second example is an investigation of crack widths in a reinforced concrete beam subjected to constant bending moment, which was tested by Braam 5. Beam No. 7 tested by Braam is selected
38、in this paper. It is a four-point bend beam with T-shape cross-section, which means that the central part is subjected to a constant moment. The calculated crack patterns with those obtained from the experiment are compared in Figure 12. In addition, it is possible to determine average crack width a
39、long the beam web (Figure 13). 5. Application to Concrete Tunnel Design This section presents an application of nonlinear analysis to a practical engineering problem, which in this case is a pre-cast reinforced concrete tunnel under a highway Pilsen-Nuernberg. The geometry of this tunnel is shown in
40、 Figure 14. The tunnel is 8 m wide and 5.6 m high with the wall thickness of 0.3 m. The tunnel walls are reinforced along inner and outer surfaces, and the tunnel is loaded with the weight of the soil filling above the tunnel, active soil pressure from both sides and a temporary loading on top of th
41、e soil filling due to a vibration machine. The finite element model, which was used in the analysis can be seen on Figure 16. It consists of 550 quadrilateral elements with 8 degrees of freedom. There are 5 elements through the wall thickness. The tunnel is built of pre-cast segments and there are c
42、onstruction joints at approximately third height of the tunnel walls. For comparison, the problem was analyzed using both linear and nonlinear finite element analyses by program SBETA (ATENA). The results are summarized in the form of moment, normal and shear force diagrams in Figures 17 to 22. Line
43、ar analyses are always on the left side and nonlinear on the right. In this way it is possible to compare the results, and observe the redistribution of internal forces due to the nonlinear behavior, which in this case can be mainly attributed to concrete cracking (Figure 15). The moment, normal and
44、 shear force diagrams are obtained automatically in the program by integration of normal and tangential stresses respectively. The stresses are integrated through the wall thickness along lines normal to the wall central axis in the finite element centers. Figure 14: Geometry and loading for the hig
45、hway tunnel.Figure 16: Finite element model with cracks from nonlinear analysisFigure 15: Cracked regions in the nonlinear analysis of the highway tunnel.This analysis demonstrates some of the advantages of nonlinear analysis for structural design. Due to the nonlinear behavior of reinforced concret
46、e where cracks can develop even for relatively low loading levels a significant stress redistribution takes place. In this case, this redistribution decreases the internal moments and increases normal and shear forces, which is a change in the favorable direction, and can result in savings of reinfo
47、rcement. Generally, in statically indeterminate structures, this redistribution does not have to be always favorable for the design, and nonlinear analysis is the only possibility how to recognize and quantify the redistribution effects. Figure 17: Moments in the tunnel walls from linear analysis.Fi
48、gure 20: Moments in the tunnel wals from nonlinear analysis.Figure 18: Normal forces in the tunnel wals from linear analysisFigure 21: Normal forces in the tunnel wals from nonlinear analysisFigure 19: Shear forces in the tunnel wals from linear analysisFigure 22: Shear forces in the tunnel wals fro
49、m nonlinear analysis6. Offshore Platform An offshore platform is a large complex structure requiring the employment of advanced design methods. The internal force distribution is statically indeterminate and thus depends on the nonlinear material behavior. As an example a simulation of the behavior
50、of the tri-cell wall from an offshore platform is presented . The failure of the tri-cell wall was the cause of the total loss of the structure as reported in ref. 4. Figure 23: Offshore platform geometryThe platform was a large cellular reinforced concrete structure as shown in Figure 23. During co
51、nstruction it undergoes submerging for deck-mating and then it is again elevated and positioned in the site. The tri-cell wall must be designed to resist the water pressure due to submerging. The analytical model is made for a symmetrical section of the tri-cell wall. The detail of joint is the same
52、 as the one in the real structure, except the wall ends, which are slightly modified to accommodate the loading forces, which substitute the action of the middle part of the wall. This geometry corresponds to the specimens for experimental investigation of the tri-cell wall 5. Two finite element mes
53、hes were used in the analysis, the coarse with 549 and the fine with the 1695 elements, respectively. The material parameters are: concrete compressive strength fc=57.4MPa, steel reinforcement yield stress fy=550 MPa. Additional parameters needed for the nonlinear constitutive law in SBETA (ATENA) w
54、ere derived form the compressive strength. Three loading forces represent the real loading conditions: 1.axial normal force in the large cell wall, 2.excentric normal force in the inclined tri-cell wall, 3.shear force normal to the tri-cell wall (representing the water pressure inside the tri-cell;
55、in the FE-model this inclined force is substituted by two orthogonal components). The relation of the amplitudes of these forces varied through the loading. The same load history as the one defined for experiments was used for the loading of FE-models. Due to the nonproportional loading the Newton-R
56、aphson iteration method had to be used. The reinforcing is shown in Figures 25 and 27. The critical shear section is reinforced by the T-headed bars. The length of the T-headed bars is important for the shear resistance. Two cases are studied: case Y2 with short bar, Y7 with long bar. The analysis w
57、as performed for both cases of reinforcement and for two FE meshes with the prescribed non-proportional loading. The loading forces are related to the water pressure and therefore, the water depth is taken as an independent measure of loading. The analysis provided a detail insight into the tri-cell
58、 behavior during the loading history, which can be used to study the structural response at any water depth. Only selected results of the failure states are shown here, and the rotated crack model is always used. The graphical documentation of the failure can be seen in Figures 24 and 26 for the cas
59、es Y2 and Y7, respectively. The failure is in both cases in the tri-cell wall under combination of normal and shear forces. The case with the short bar fails at the water depth 70m, Figure 24. The failure plane bypasses the bar and the failure is mainly due to concrete fracture. The case with the lo
60、ng bar fails at the water depth 110m, Figure 26. The bar is activated and the shear failure is not concentrated into one narrow plane. The failure is due to the reinforcement yielding and the concrete fracture. Evidently, the longer T-headed bar increases the shear resistance of the wall. Similar re
61、sults were obtained for the coarse meshes, but their graphical results are not presented here. The load-displacement diagrams are compared in Figure 28. The water level obtained in experiments 5 are also shown. The failure loads from computer simulations agree well with experimental results. The fin
62、e and coarse mesh solutions give very similar results until the shear failure crack forms. Behavior near the peak load is more influenced by the mesh size, which is probably due to the brittle behavior, and the fine mesh gives consistently lower response. However, the difference is not too large. Th
63、e response curve has only ascending branch due to the use of the Newton-Raphson method. Thus, the flat plateau near the peak load need not to reflect the correct response of the structure. In reality, the response can be more brittle, with a descending branch. Such response could be simulated numeri
64、cally if an alternative loading scheme is considered. Figure 24: Crack pattern with concrete crushing for analysis with short barFigure 26: Crack pattern with concrete crushing for analysis with long barFigure 25: Reinforcement yielding for analysis with short barFigure 27: Reinforcement yielding fo
65、r analysis with long bar4. CONCLUDING REMARKS The nonlinear finite element analysis is an advanced tool for design of reinforced concrete structures. If based on the smeared crack model, which is a well accepted approach, it should meet certain requirements in order to ensure a safe application. On the constitutive level the material model must be able to reproduce the failure modes important for the structural behavior under consideration. In concrete structures with extensive crack propagation the constitutive law has to describe the nonlocal
- 温馨提示:
1: 本站所有资源如无特殊说明,都需要本地电脑安装OFFICE2007和PDF阅读器。图纸软件为CAD,CAXA,PROE,UG,SolidWorks等.压缩文件请下载最新的WinRAR软件解压。
2: 本站的文档不包含任何第三方提供的附件图纸等,如果需要附件,请联系上传者。文件的所有权益归上传用户所有。
3.本站RAR压缩包中若带图纸,网页内容里面会有图纸预览,若没有图纸预览就没有图纸。
4. 未经权益所有人同意不得将文件中的内容挪作商业或盈利用途。
5. 装配图网仅提供信息存储空间,仅对用户上传内容的表现方式做保护处理,对用户上传分享的文档内容本身不做任何修改或编辑,并不能对任何下载内容负责。
6. 下载文件中如有侵权或不适当内容,请与我们联系,我们立即纠正。
7. 本站不保证下载资源的准确性、安全性和完整性, 同时也不承担用户因使用这些下载资源对自己和他人造成任何形式的伤害或损失。