soilpileinteractioneffects
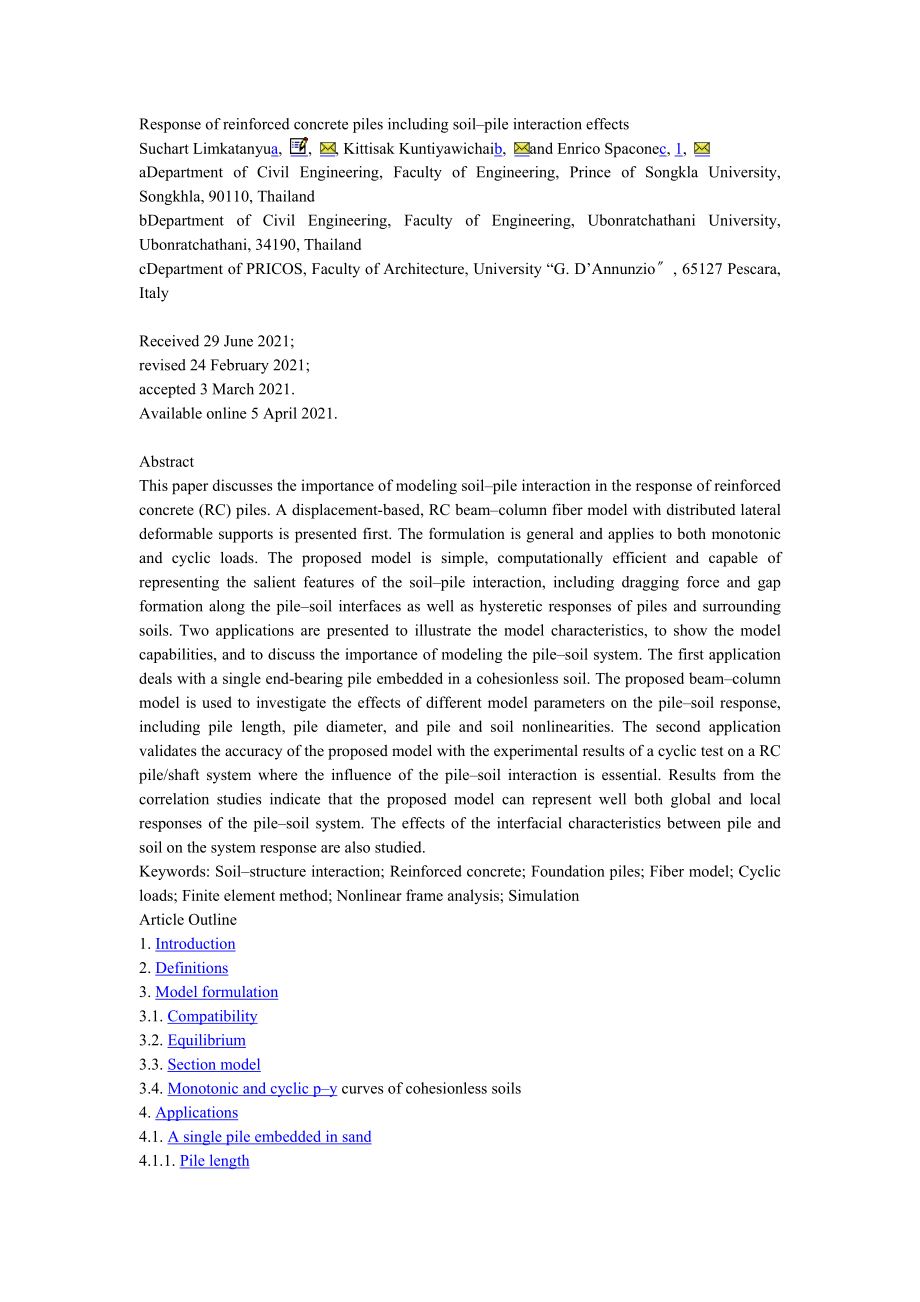


《soilpileinteractioneffects》由会员分享,可在线阅读,更多相关《soilpileinteractioneffects(24页珍藏版)》请在装配图网上搜索。
1、Response of reinforced concrete piles including soilpile interaction effects Suchart Limkatanyua, , , Kittisak Kuntiyawichaib, and Enrico Spaconec, 1, aDepartment of Civil Engineering, Faculty of Engineering, Prince of Songkla University, Songkhla, 90110, ThailandbDepartment of Civil Engineering, Fa
2、culty of Engineering, Ubonratchathani University, Ubonratchathani, 34190, ThailandcDepartment of PRICOS, Faculty of Architecture, University “G. DAnnunzio, 65127 Pescara, ItalyReceived 29 June 2021; revised 24 February 2021; accepted 3 March 2021. Available online 5 April 2021. AbstractThis paper di
3、scusses the importance of modeling soilpile interaction in the response of reinforced concrete (RC) piles. A displacement-based, RC beamcolumn fiber model with distributed lateral deformable supports is presented first. The formulation is general and applies to both monotonic and cyclic loads. The p
4、roposed model is simple, computationally efficient and capable of representing the salient features of the soilpile interaction, including dragging force and gap formation along the pilesoil interfaces as well as hysteretic responses of piles and surrounding soils. Two applications are presented to
5、illustrate the model characteristics, to show the model capabilities, and to discuss the importance of modeling the pilesoil system. The first application deals with a single end-bearing pile embedded in a cohesionless soil. The proposed beamcolumn model is used to investigate the effects of differe
6、nt model parameters on the pilesoil response, including pile length, pile diameter, and pile and soil nonlinearities. The second application validates the accuracy of the proposed model with the experimental results of a cyclic test on a RC pile/shaft system where the influence of the pilesoil inter
7、action is essential. Results from the correlation studies indicate that the proposed model can represent well both global and local responses of the pilesoil system. The effects of the interfacial characteristics between pile and soil on the system response are also studied.Keywords: Soilstructure i
8、nteraction; Reinforced concrete; Foundation piles; Fiber model; Cyclic loads; Finite element method; Nonlinear frame analysis; SimulationArticle Outline1. Introduction 2. Definitions 3. Model formulation 3.1. Compatibility 3.2. Equilibrium 3.3. Section model 3.4. Monotonic and cyclic py curves of co
9、hesionless soils4. Applications 4.1. A single pile embedded in sand 4.1.1. Pile length 4.1.2. Pile diameter 4.1.3. Soil and pile nonlinearities4.2. ChaiHutchinson pile #15. Summary and conclusions Acknowledgements References1. IntroductionDue to the increasing need for infrastructures and the decrea
10、sing availability of space, both structural and geotechnical engineers are challenged to design, analyze, and evaluate more expensive and strategic structural systems (e.g.high-rise buildings, offshore platforms, multi-story highways, etc.) for extreme lateral loadings (e.g.earthquakes, gusty winds,
11、 terrorist attacks, etc.). In weaker soils, foundation piles, both single pile and pile groups, may be used to transfer large superstructure loads through deeper soils. In these cases, the influence of the foundation piles should be included. Typically, seismic design codes (e.g.NEHRP-971) neglect t
12、he seismic behavior of the foundation piles, or they greatly simplify it, because the structural-period lengthening due to the foundation flexibility for most design spectra leads mainly to a reduction in seismic design forces. This is not always the case when near-fault ground motions (possibly con
13、taining long-period pulses) are considered or when the structural displacements rather than the structural forces are the key design parameters, as in the case of the Displacement-Based Seismic Design Methodology2 and 3. Furthermore, damages of the pile foundations during recent destructive earthqua
14、kes (e.g.1989 Loma Prieta Earthquake4, 1995 Hyogoken-Nambu Earthquake5 and 6, etc.) are reminders of the importance of the foundation piles and of their influence on the overall structural response. Consequently, the inclusion of the soilpile system into the foundation numerical model may be importa
15、nt for design, analysis, and performance evaluation of structures under seismic actions. It is commonly accepted that foundation piles should remain elastic under seismic loadings in order to avoid difficult subsurface inspections and expensive repairs of the damaged foundations. Nevertheless, the b
16、ending moment induced in the piles by the design seismic actions can be large enough to cause flexural damage in the piles, as shown by recent post-earthquake investigations4, 5 and 6. The flexural damage in the piles can reduce both the foundation stiffness and its strength, hence affecting the ser
17、viceability and load-carrying capacity of the whole structurefoundationsoil system. Furthermore, the role of the foundation piles in the seismic rehabilitation of existing buildings is crucial. Seismic retrofit of existing buildings can be economically achieved by the adding steel bracing or RC shea
18、r walls. This can however result in severe seismic demands on the existing foundation piles. Thus far, several analytical models have been proposed in the published literature to study the soilpile interaction problem. These models range from comparatively simple approaches in which the soils surrou
19、nding the pile are represented as a set of discrete springs, to sophisticated 3D finite element models. These analytical models are classified here into three categories: (a) elastic continuum models7; (b) solid (2D or 3D) finite element models8, 9, 10, 11 and 12; (c) line (1D) beamcolumn elements b
20、ased on beam theories13, 14 and 15. The pros and cons of the aforementioned analytical models are summarized in Limkatanyu and Spacone16. The boundary element models have also been used to study the soilpile interaction problem17.The main objectives of this paper are to present a newly developed pil
21、esoil beamcolumn element, to study its main parameters, and to validate the proposed model through correlation studies of large-scale tests on piles subjected to lateral loadings. The formulation of the pilesoil beamcolumn element is based on the principle of virtual displacements. Other element for
22、mulations are presented elsewhere16. The Winkler Foundation model is employed to represent the surrounding soils (Winkler18). Two applications are presented to illustrate the model characteristics, to show the model capabilities, and to discuss the importance of modeling the pilesoil system. The fir
23、st application investigates the effects of several model parameters on the lateral response of the pilesoil systems. The second application deals with the correlation studies of a large-scale RC pile/shaft experimental test. It is important to note that this study focuses only on the soilpile intera
24、ction in firm non-liquefiable soils. The kinematic interaction due to scattering of the incoming seismic waves and the soil liquefaction effects are not included in this study.2. DefinitionsThe 2-node displacement-based beamcolumn element with lateral deformable supports (e.g.soil)16 is shown in Fig
25、.1. The proposed beamcolumn element with lateral deformable supports comprises two components: a 2-node beamcolumn, plus continuous lateral springs representing the surrounding deformable medium.Full-size image (36K)Fig.1.2-node displacement-based beamcolumn element with lateral deformable supports1
26、6. View Within ArticleUsing the notation of Fig.1, the element nodal displacements are: (1)where and are arrays containing the displacements at nodes 1 and 2, respectively. The corresponding nodal forces are . The section displacements are grouped in the following array: (2)where uB(x) and vB(x) rep
27、resent the axial and transverse displacements, respectively. The section deformations are grouped in the following array: (3)where B(x) and B(x) are the axial strain and the curvature, respectively. The beamcolumn formulation follows the EulerBernoulli beam theory. Based on the small deformation ass
28、umption, the section deformations are found through the compatibility relations and . In matrix notation: (4)where B is the following differential operator: (5)The corresponding section forces are: (6)where NB(x) and MB(x) are the axial load and the bending moment, respectively. The lateral deformat
29、ions are defined by the following array: (7)where and are the lateral deformations at the top and bottom faces, respectively. Based on the Winkler foundation theory, the lateral deformations are determined through the following compatibility relations: (8)Though these displacements are equal in the
30、present formulation, they are kept distinct to maintain generality. Eq. (8) can be expressed in the following matrix form: (9)where is a transformation matrix defined as: (10)Finally, the lateral forces and corresponding to the lateral-support deformations are grouped in the array: (11)3. Model form
31、ulationThe element formulation is based on the principle of virtual displacements. The element displacements are expressed as functions of the nodal displacements U through the shape functions : (12)are well-known Hermitian polynomials that define a linear axial displacement field and a cubic latera
32、l displacement field. 3.1. CompatibilityCompatibility is enforced in the strong form. Upon substitution of Eq. (12) into Eqs. (4) and (9), the section and lateral deformations are directly related to the nodal displacements U through the following equations: (13)where is the pile section deformation
33、displacement matrix and is the soil deformationdisplacement matrix. 3.2. EquilibriumElement equilibrium is enforced in the weak form. Application of the principle of virtual displacements and substitution of Eq. (13) yield the following equation: (14)The total internal forces are expressed as the su
34、m of the initial section forces and plus the force increments and . is the beam section stiffness matrix and is the matrix containing the lateral-support stiffness. Then: (15)Eq. (15) is rearranged to obtain the following incremental form of the equilibrium: (16)where K is the element stiffness matr
35、ix and is the element force array (17)(18)is the array containing the element initial forces. In Eq. (17), is the beamcolumn contribution to the element stiffness, and is the contribution of the lateral supports: (19)Similarly, in Eq. (18), is the beamcolumn contribution to the element forces, and i
36、s the contribution of the lateral supports: (20)3.3. Section modelThe cross section is subdivided into fibers. The beam section forces are (21)where j represents the generic fiber and nfib is the number of fibers in the RC section. yj, j, and Aj are the distance from the reference axis x (Fig.1), th
37、e stress, and the area, respectively, of fiber j of the beamcolumn section. Indicating with Ej the fiber modulus, the beamcolumn section stiffness is (22)The lateral-support forces are: (23)where and are the lateral pressures at the top and bottom interfaces, respectively. D is the width of the beam
38、column section. The lateral-support stiffness matrix is a diagonal matrix defined as: (24)and are the moduli of the top and bottom lateral supports, respectively. The hysteretic behaviors of the concrete and steel laws are schematically presented in Fig.2. The Kent and Park19 law is used for the con
39、crete. The tensile branch of the concrete is not considered in the present study. The Menegotto and Pinto20 law is used for the steel reinforcement. For the present study, the finite element program FEAP21 is used to host the proposed element.Full-size image (41K)Fig.2.Hysteretic uniaxial material m
40、odels: (a) Concrete; (b) Steel reinforcement. View Within Article3.4. Monotonic and cyclic py curves of cohesionless soilsIn this study, the soils surrounding a pile are modeled as 1D springs continuously distributed along the pile length. Only cohesionless soils are considered in this study. Based
41、on the model proposed by Reese etal.22, the ultimate soil lateral strength pu can be computed as the lesser of the following two values: (25)(26)where Kp=tan2(45+/2) is the passive earth pressure coefficient; Ka=tan2(45/2) is the active earth pressure coefficient; K0 is the at-rest earth pressure co
42、efficient; is the effective internal friction angle; =/2 is the angle defining the failure wedge shape; =45+/2; is the soil effective unit weight; z is the depth from the ground surface; and D is the pile diameter. The typical value of the at-rest earth pressure coefficient K0 is 0.4, as recommended
43、 by Reese and Van Impe23. Eq. (25) accounts for the wedge-type failure near the surface while Eq. (26) accounts for the plane-strain failure at great depths below the ground surface. The monotonic backbone py curves relating the soil deformation to the soil pressure are shown for various depths in F
44、ig.3. Each monotonic backbone py curve comprises three linear portions and one parabolic portion. The initial linear portion (oa) represents the elastic behavior of the soil for small lateral deflections as suggested by Terzaghi24. The slope of this linear portion is equal to the product of the modu
45、lus subgrade nh and the depth z measured from the surface. The recommended values of nh can be found in Reese and Van Impe23. The flat-top portion (beyond c) represents the plastic behavior of the soil for large lateral deflections. These two linear portions are connected by a parabola (ab) and an i
46、nclined straight line (bc) in order to obtain a shape consistent with the experimental py curves22. It is noted that the deeper soils are stiffer and stronger than the shallower soils due to the high confining pressures. Full-size image (28K)Fig.3.Characteristic shape of py curves for sand (proposed
47、 by Reese etal.22). View Within ArticleThe monotonic py curves of Fig.3 serve as envelopes of the two cyclic py shown in Fig.4. The model of Fig.4(a) is elastic-perfectly plastic and ignores the gapping and dragging responses of the surrounding soil. The model of Fig.4(b) takes into account the gapp
48、ing and dragging responses observed during in situ tests on piles under lateral cyclic loadings (e.g.Brown etal.25). The hysteretic characteristics of the model developed by Arnold etal.26 are modified for the proposed cyclic py model (Fig.4(b). The soil is loaded first along the monotonic branch oa
49、 and then is elastically unloaded with the initial stiffness until it reaches the dragging strength at pd at point b in Fig.4(b). As unloading is reloading and reloading continues in the opposite direction, the soil moves through the gap until it reaches the monotonic envelope on the opposite side a
50、t point c. A sixth-order polynomial is used to connect points b and c to model the gap-closing process. The same scenario of the unloadingreloading process is repeated for the branch dea. The dragging force is activated only when the pile moves through the gap. The value of the dragging strength pd
51、is taken as 30% of the monotonic peak capacity pu, as suggested by Hutchinson etal.27.Full-size image (30K)Fig.4.Hysteretic py models: (a) Elastic-perfectly plastic model; (b) Dragginggapping model. View Within Article4. ApplicationsTwo applications were selected to investigate the model parameters,
52、 to show the model capabilities, and to discuss the importance of modeling the pilesoil sub-system. The first one is a purely numerical example used to test the relevance of several model parameters through a set of parametric studies. The second one considers the experimental results of a cyclic te
53、st on a RC pile/shaft system where the influence of the pilesoil interaction is deemed essential.4.1. A single pile embedded in sandThe pilesoil system shown in Fig.5 is used to investigate the effects of the model parameters on the system response. Thirty beamcolumn elements are used to model the p
54、ilesoil system. Such a refined mesh is used to ensure that the converged solution is reached. The model parameters investigated include the pile length, the pile diameter, and the pile and soil nonlinearities.Full-size image (36K)Fig.5.Numerical example: An end-bearing pile embedded in sand. View Wi
55、thin Article4.1.1. Pile lengthIn this section, the pile response is kept linear elastic and the py curves of Fig.3 are used to represent the responses of the surrounding sand. The pile-length effects on the lateral behavior are investigated by keeping the pile diameter constant at 0.5 m and varying
56、the pile length from 5 to 15 m. Consequently, the pile slenderness ratios (L/D) range from 10 to 30. The pile-length effects on the global response are presented in the form of the lateral loaddeflection curves in Fig.6. The pile-length effects on the local responses are shown through the pile defle
57、cted shapes in Fig.7 and the moment distributions along the pile in Fig.8. These responses refer to a pile-head deflection of 0.15 m.Full-size image (31K)Fig.6.Lateral loaddisplacement pile responses for different pile lengths. View Within ArticleFull-size image (28K)Fig.7.Deflected curves for diffe
58、rent pile lengths (for a head displacement ). View Within ArticleFull-size image (32K)Fig.8.Moment distributions along the pile for different pile lengths (for a head displacement ). View Within ArticleThe pile length has little effect on the global response at the early loading stages. The explanat
59、ion can be found by observing the pile deflected shapes in Fig.7. Irrespective of the pile length, the top 3 m deform approximately the same way. The deflections of the rest of the pile, however, vary. The 5 m and 6.25 m long piles act as poles instead of piles. The tip deflections of the short pile
60、 are significant, hence resulting in yielding of the soils surrounding the pile tips. Conversely, the additional length of the longer pile acts as anchor and thus stiffens the pile.Additionally, it can be observed that both global and local responses converge when the pile length is larger than 7.5
61、m (L/D=15). In other words, the pile development length is approximately 7.5 m. This phenomenon is similar to the development length of the reinforcing steel in RC structural elements. For an elastic pile embedded in an elastic cohesionless soil, the characteristic length of the elastic soilpile sys
62、tem is 28. This value is smaller than the observed development length of 7.5 m due to the fact that the characteristic length increases when the soil becomes nonlinear. It is observed in Fig.8 that the maximum bending moment location is approximately the same for different pile lengths.4.1.2. Pile d
63、iameterIn this section, the pile response is still kept linear elastic and the py curves of Fig.3 are used to represent the response of the surrounding sand. The pile-diameter effects on the lateral behavior are assessed by keeping the pile length constant at 15 m and varying the pile diameter from
64、0.5 to 1.5 m. Consequently, the pile slenderness ratio (L/D) varies between 10 and 30. The pile-diameter effects on the global responses are presented in the form of the lateral loaddeflection curves in Fig.9. The pile-diameter effects on the local responses are shown by the pile deflected shapes in Fig.10 and by the moment distributions along the pile in Fig.11. These loca
- 温馨提示:
1: 本站所有资源如无特殊说明,都需要本地电脑安装OFFICE2007和PDF阅读器。图纸软件为CAD,CAXA,PROE,UG,SolidWorks等.压缩文件请下载最新的WinRAR软件解压。
2: 本站的文档不包含任何第三方提供的附件图纸等,如果需要附件,请联系上传者。文件的所有权益归上传用户所有。
3.本站RAR压缩包中若带图纸,网页内容里面会有图纸预览,若没有图纸预览就没有图纸。
4. 未经权益所有人同意不得将文件中的内容挪作商业或盈利用途。
5. 装配图网仅提供信息存储空间,仅对用户上传内容的表现方式做保护处理,对用户上传分享的文档内容本身不做任何修改或编辑,并不能对任何下载内容负责。
6. 下载文件中如有侵权或不适当内容,请与我们联系,我们立即纠正。
7. 本站不保证下载资源的准确性、安全性和完整性, 同时也不承担用户因使用这些下载资源对自己和他人造成任何形式的伤害或损失。
最新文档
- 市场营销沟通与促销策略教学课件2
- 市场营销活动策划及执行教学课件
- 福清美食鉴赏资料课件
- 小学语文S版四年级(下册)滥竽充数课件
- 粉体表面处理技术规范课件
- 小学语文S版三年级(下册)语文百花园二课件
- 小学语文S版三年级下册知识清单课件第五单元
- 市场营销概论-课件
- 小学道德与法治(部编版)《我的家庭贡献与责任》优质ppt课件
- 小学语文S版四年级上册知识清单课件第三单元
- 小学常规教学工作与教学改革课件
- 粉末性能及其测定课件
- 福建七年级生物上册-6.1种子萌发形成幼苗(第1课时-种子的结构)讲义-(新版)北师大版课件
- 小学语文S版六年级下册归类复习课件之现代文阅读【三】说明文、简单议论文
- 疯狂三天三夜增员宣导稿具体举措课件